Summary of a presentation by UC Davis graduate student Jordan Hollarsmith.
James Hutton, one of the first geoscientists, developed the principle of uniformitarianism, a guiding theory of the geological sciences: the processes we observe today are the same as those that occurred millions (or billions) of years ago. But how can scientists compare what they observe today to what was happening so long ago? The study of ancient fossils and plants, a discipline called paleoecology, provides a road map between our observations of the current natural world and what our planet looked like millions of years ago. The Grand Canyon in Arizona is an ideal natural laboratory for the study of paleoecology.
Rocks of the Grand Canyon span billions of years of Earth history, and the patterns scientists observe in the formations can help to unravel secrets of deep time. The Grand Canyon has been subjected to a variety of tectonic events from the earliest time the rocks were deposited, including the intrusion of igneous rocks that make up the “basement” of the canyon today and the initiation of faults that have been active at various times for the past 1 billion years. However, the paleoecology of the Grand Canyon has been most heavily influenced by changes in sea level over the course of geologic time. These sea level changes are reflected in the rock units of the canyon as compositional differences and fossil traces left behind by the flora and fauna that lived in these ancient environments.
In order to understand the types of environments that existed during earlier periods of Earth’s history, paleoecologists compare the compositions and sedimentary features of ancient rocks to the types of deposits seen in modern environmental settings. For example, the fine-grained shales that compose the Bright Angel Shale indicate that the depositional environment of the time (~515 million years ago) was likely low-energy and deep beneath the surface of the ocean. Carbonates, such as those exposed in the Redwall Limestone (deposited ~340 million years ago), are indicative of shallow marine settings only 10s of meters deep, where organisms that photosynthesize for survival can access sunlight. Terrestrial deposits, such as the Surprise Canyon Formation (deposited ~320 million years ago), were deposited in rivers or along shorelines. The large variation in particle sizes of these rock typesreveal changes in energy of the depositing waters, such as migration of rivers over time.
Fossils also contribute to our understanding of the paleoenvironments preserved in rock formations. One of the most exceptional examples of fossil preservation in the Grand Canyon is the Coconino Sandstone. Unlike other units, the Coconino Sandstone is thought to have been deposited subaerially. Cross-bedding of the sandstone formation indicates that the unit was likely deposited as sand dunes, similar to the Sahara Desert of northern Africa. Animals similar to the reptiles we see today scampered across these dunes, leaving behind their tracks. Paleoecologists are able to study the tracks left behind in these dunes due to occasional water inundation, possibly by rain or flooding, which prevented the tracks from being blown away.
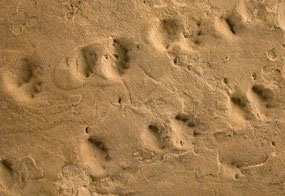
After paleoecologists have interpreted the environment that led to deposition of an observed rock unit, how do they determine when, precisely, these environments existed? This can be a very difficult problem to solve, and paleoecologists approach this issue using a variety of methods. A common method that can be applied easily by a knowledgeable scientist is comparison of specific fossil types with what is known to have appeared at other locations. If the fossils in other locations have been dated, then the correlation of known units with the units under observation can help constrain the age of the rock unit. An excellent example of such an “index fossil” is the trilobite. Trilobites diversified during the Cambrian Period (~542 million years ago) and thrived in marine environments until the Permian Extinction (~251 million years ago). Though their existence spans the entire Paleozoic Era, the identification of different trilobite species can help a paleoecologist determine the time of deposition of the rock unit containing the fossil.
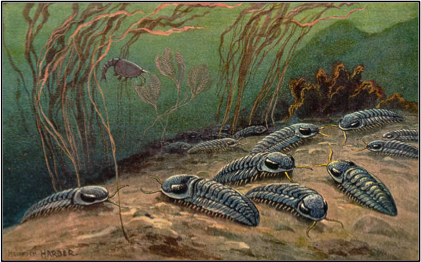
Absolute dating methods are also available to paleoecologists if the appropriate materials are available for sampling. The most commonly used method for absolute dating of rocks of Grand Canyon age is Uranium–Lead (U-Pb) dating of detrital zircons. Zircons crystallize in felsic (high-SiO2) igneous rocks at high temperatures; once they’re formed, they’re very difficult to destroy! After being eroded out of their igneous host, the zircon grains may travel great distances before being deposited in sedimentary units. Subsequent dating by paleoecologists yield a maximum age of deposition: because the zircons are of a certain age, the sedimentary unit cannot be older than the zircons it contains.
By using the various tools available to paleoecologists, we can understand the environments that existed millennia before humans walked the Earth and compare them to what we see today.