Starting in 1934, millions of Tamarix were planted throughout Texas, Oklahoma, Kansas, Nebraska, and North and South Dakota as part of the Great Plains Shelterbelt project, in an attempt to slow the wind-erosion of topsoil occurring in the disastrous Dust Bowl. By 1938 Tamarix had spread and become established along the Colorado River in the Grand Canyon (Jotter & Clover 1944) and along the Green River (Birken & Cooper 2006), the largest tributary to the Colorado. Tamarix is now widespread across several million acres of land in the western US and continues to expand along river corridors in both dam-regulated and unregulated reaches (Perkins, Scott & Naumann 2015).
Invasive species have had well-documented impacts to native biodiversity in many locations around the world via predation, competition, parasitism, and/or habitat or ecosystem process modification (Pimentel, Zuniga & Morrison 2005; McGeoch et al. 2010). However, many non-native species integrate into native ecosystems without having quantifiably significant negative impacts (Daehler & Carino 2000), or are even intentionally introduced for their beneficial effects (Howarth 1991), which can sometimes lead to unforeseen negative outcomes (Louda 1997). However, the terminology surrounding non-native species is not standardized (Colautti & MacIsaac 2004), and so labels such as “invasive”, “weed”, and “noxious” are often attached to non-native species without significant supporting science documenting their implied negative impacts to native ecosystems and resources. Because invasive species can be extremely difficult to eradicate once established, it is prudent and cost- effective to accurately identify which species are currently, or are likely to be, causing harm to ecosystems (Keller, Lodge & Finnoff 2007).
In addition to direct and quantifiable harm to native species or ecosystem services, non- native species introductions are often seen as undesirable in their own right, regardless of measurable impact. The presence of non-native species, in the opinion of some people or public agencies, significantly detracts from the historic nature or aesthetics of a place simply by being there. One such agency is the National Park Service (NPS), which has a mandate to manage natural systems in their “unimpaired state” state. The NPS attempts to prevent new non-native species from entering Parks, while eradicating or containing existing invasions. However, most land owners and managers (other federal agencies, states, private entities, etc.) do not have such a clear mandate with regard to naturally functioning ecosystems. For them, the decision to expend resources dealing with a non-native species must often be justified in terms of mitigating a measurable impact that the non-native is having on a protected species or valuable resource.
Because non-native species management is costly, it is imperative to target control efforts and limited resources in an efficient manner. One critical tool for managers is sound science that provides an ecological understanding of the target organism and its interaction with environmental processes and the native community into which it has established. In addition to informing a management or restoration strategy, careful science may be able to provide additional justification or impetus for control of a non-native by quantifying its impacts on other species or resources.
In the southwestern United States, many non-native plants have become established along large rivers flowing through arid landscapes. One of the most widespread, well-known, and highly managed species is Tamarix ramosissima (actually a hybrid species-complex with Tamarix chinensis, (Gaskin, Birken & Cooper 2012)), hereafter referred to as Tamarix (but also known as tamarisk or salt cedar). Starting in 1934, millions of Tamarix were planted throughout Texas, Oklahoma, Kansas, Nebraska, and North and South Dakota as part of the Great Plains Shelterbelt project, in an attempt to slow the wind-erosion of topsoil occurring in the disastrous Dust Bowl. By 1938 Tamarix had spread and become established along the Colorado River in the Grand Canyon (Jotter & Clover 1944) and along the Green River (Birken & Cooper 2006), the largest tributary to the Colorado. Tamarix is now widespread across several million acres of land in the western US and continues to expand along river corridors in both dam-regulated and unregulated reaches (Perkins, Scott & Naumann 2015).
Tamarix has been the focus of intensive management throughout the western US. In 2001 an insect called the northern tamarisk beetle (Diorhabda carinulata) was introduced intentionally as a biological control agent. The beetle is a leaf herbivore on Tamarix, and co- occurs with the plant in their native ranges in central Asia. However, this species is not well adapted to all of the western US climates that Tamarix has colonized, so three other closely- related beetle species (D. elongata, D. carinata, and D. sublineata) have also been introduced to distinct subregions of the West (Tracy & Robbins 2009). The beetles are thriving and have spread through 13 western states and now extend from Mexico to Montana, California to Oklahoma (Tamarisk Coalition 2015).
In addition to the introduction of four non-native taxa as biological controls agents, millions of dollars have been spent on physical removal, herbicide treatment, and burning of Tamarix. A Federal law called the “Salt Cedar and Russian Olive Control Demonstration Act”, passed in 2006, allocated $80 million over a 5-year period for removal and treatment of the two eponymous species. Part of the goal of this demonstration was to quantify the impact that Tamarix (and Russian olive) have on resources and native ecosystems and how removal efforts can reverse those impacts. Early versions of the Act introduced to committees in Congress in 2003 contained language citing impacts as justification for the proposed effort:
“[Tamarix] is a nonbeneficial user of 2,000,000 to 4,500,000 acre-feet of water per year; the amount of nonbeneficial use of water by tamarisk is greater than the amount that valuable native vegetation would have used; and represents enough water for-- (i) use by 20,000,000 or more people; or (ii) the irrigation of over 1,000,000 acres land.
Scientists have established that tamarisk infestations can--
(A) increase soil and water salinity;
(B) increase the risk of flooding through increased sedimentation and decreased channel conveyance;
(C) increase wildfire potential;
(D) diminish human enjoyment of and interaction with the river environment; and
(E) adversely affect--(i) wildlife habitat for threatened and endangered species; and (ii) the abundance and biodiversity of other species.
As drought conditions and legal requirements relating to water supply accelerate water shortages, innovative approaches are needed to address the increasing demand for a diminishing water supply.”
As outlined in a review article (Stromberg et al. 2009), most of these or similar claims have little or no scientific basis. The assumed impacts (often misattributed as scientific facts) of Tamarix on water supply, soil salinity, and native riparian plant communities, are often repeated to this day, as is the assumption that river regulation downstream of dams has caused or exacerbated the expansion of Tamarix. However, numerous recent studies have begun to address these assumptions using scientific methods, and are now offering a reality-based picture of the causes and effects of Tamarix establishment. For example, recent assessments of the potential for water savings by removing Tamarix now range between 30,000 acre feet per year for the entire Colorado River basin (Bureau of Reclamation 2012), to an acknowledgement that the true value is highly uncertain, but not likely to be much greater than zero (Sher & Quigley 2013). Therefore, the current scientific consensus is that uncertain, but probably very small, increases in water yield can be expected from large-scale Tamarix removal.
A primary, and often stated, assumption underlying the management of Tamarix is that its spread was caused by flow regulation after the construction of large dams on the Colorado River and its major tributary, the Green River. Understanding whether or not this is true is essential for developing a management and restoration plan, because if Tamarix is only highly successful at colonizing regulated rivers, natural-flowing rivers should be highly resistant to invasion, and controlled floods should be an effective management technique. However, ample scientific evidence has recently been gathered to show that 1) Tamarix initially colonized and proliferated on the Green and Colorado Rivers decades before dam construction (Birken & Cooper 2006), and 2) the current spread of Tamarix is proceeding up unregulated rivers such as the Yampa (Merritt & Poff 2010).
Although Hoover Dam began regulating flow on the Colorado River in 1935, it affects only the last ~350 miles of the river before it enters the ocean. Above Hoover Dam, the other ~1,100 miles of the Colorado River, and its largest tributary, the 730 mile-long Green River, remained unregulated until 1962-3, when the Glen Canyon and Flaming Gorge dams began impounding water, effectively regulating flow along almost the entire length of those two rivers. Observations in the Grand Canyon (Jotter & Clover 1944) and dendrochronolgic, historic photograph and floodplain stratigraphic analyses on the Green River (Birken & Cooper 2006) show that Tamarix colonized and expanded dramatically in the unregulated rivers above Hoover Dam between 1938 and 1962. This colonization of unregulated rivers continues at present, with Tamarix establishing in mixed stands of native riparian woody species such as cottonwood (Populus sp.) (Merritt & Poff 2010).
Because it is clear that Tamarix historically and currently form mixed stands within native plant communities, it is important to understand the competitive relationships between these species. A set of oft-repeated assumptions claim that Tamarix is a superior competitor with native woody riparian plants, and that it also has allelopathic capacity via salination of the soil in which it grows. Claims of allelopathy and soil salination have been based on correlative studies showing Tamarix tolerance for salty sites, not its alteration of a site towards greater salinity (see Stromberg et al. 2009). Recent studies have shown that soil and leaf litter under Tamarisk enhanced growth of wheatgrass and showed no signs of allelopathy (Lesica & DeLuca 2004) and that soil under Tamarix in China had elevated fertility (organic matter and nutrients) as well as elevated salinity as compared to adjacent bare ground (Yin et al. 2010). There is no conclusive or credible evidence that Tamarix has significant allelopathic effects on vegetation. Although some soil salination may occur, especially in sites with already-salty water (several of the Chinese study sites have naturally salty groundwater), the extent and magnitude of this effect within the Colorado River basin is completely unknown.
Several direct studies of competition between Tamarix and native woody riparian species have shown that native seedlings and adults are equal or superior competitors. An investigation into competition for water between adult Tamarix and native cottonwood, ash, and mesquite found that Tamarix was no better at competing for water than natives, suggesting that mixed native/non-native stands would be persistent and not become dominated by Tamarix (McGuire 2015). In a seedling growth experiment Populus outcompeted Tamarix across all sediment-type and hydrology treatments, whereas Tamarix only negatively impacted Populus at already-low Populus densities (Sher & Marshall 2003). Another seedling experiment found that both Tamarix and Populus seedings were killed by simulated-flood sediment burial, but that native box elder seedlings were able to push up through overlying sand and survive (Kui & Stella 2016). A multi-faceted study looking at shade tolerance of Tamarix and box elder seedlings and Tamarix adults demonstrated that box elder seedlings have greater shade tolerance and that Tamarix adults are killed after 1-2 seasons of shade, simulating canopy coverage by the taller box elder (Dewine & Cooper 2008).
These studies demonstrate that Tamarix colonized (and is currently still colonizing) naturally-flowing rivers, integrating into the native plant community. It is also emerging that native woody riparian plants, at both the seedling at adult stages, are equal or superior competitors with Tamarix, and that it is unlikely that Tamarix establishment alone causes significant displacement of native woody plant communities. However, there is also a large body of literature demonstrating that river regulation severely limits native seedling establishment. Tamarix has a much broader seed dispersal period than natives. So, although it has better establishment success on high flow years (Birken & Cooper 2006), its prolonged seed dispersal allows it to take advantage of small opportunities even on low flow years or on regulated rivers. This is in contrast to native woody plants, whose seed dispersal is limited to spring, in conjunction with the timing of the recession of peak flow. Where high flow events no longer occur, the synchronous rain of native seeds is unlikely to fall on the required bare moist substrate. Current evidence suggests that the primary limitation on native plant establishment is flow regulation, not competition from Tamarix. The appearance of Tamarix domination along regulated rivers is more a function of flow-inhibition of native establishment (and Tamarix tolerance of low flows) rather than Tamarix directly outcompeting native plants (Merritt & Poff 2010). By contrast, the mixed stands of Tamarix and native plants along unregulated rivers indicate the success of natives and non-native alike in the presence of a natural flow regime.
It is now thought that Tamarix would have spread and colonized much of its current range even if no dams had been built (Merritt & Poff 2010). So, although the dams did not cause the spread of Tamarix, flow regulation is clearly suppressing native plant establishment by a greater degree than Tamarix (Figure 1). As a result, simply removing Tamarix along regulated reaches is unlikely to significantly increase native woody plant establishment on its own. Either high spring flow dam releases, and/or active planting of native species will be required. Once given an opportunity to establish, native plants are likely to be successful competitors even within new or existing Tamarix stands.
This set of ecosystem studies can be used to formulate new management recommendations that focus on assisting native plant establishment (primarily impacted by flow regulation). This is in contrast to the previous management strategy that focused on removing Tamarix, which has not been shown to have nearly the impacts it was assumed to have. However, there are still likely to be areas where direct removal of Tamarix is desirable, such as in Grand Canyon National Park. Because part of the Park’s mandate is to remove non- native species, which are seen as an aesthetic impact in their own right, direct removal of Tamarix within the Grand Canyon will still need to occur. Ironically, the introduced tamarisk beetle has colonized the Grand Canyon, adding at least one more non-native species to the Park. However, it is hoped that the beetle, in conjunction with physical removal might achieve complete eradication of Tamarix in the Park. This will be a Herculean task, and recolonization will be a constant threat, but the prospect of removing a non-native from a National Park may be worth the cost. Outside of National Parks, the lack of such a clear mandate on non-natives means that other benefits of removal will probably have to be shown to justify the immense cost of eradication, likely to be in the many billions of dollars. Further science may demonstrate those benefits, but unsupported assumptions of the past have started to crumble, and should no longer be relied on as the foundation of a Tamarix management plan.
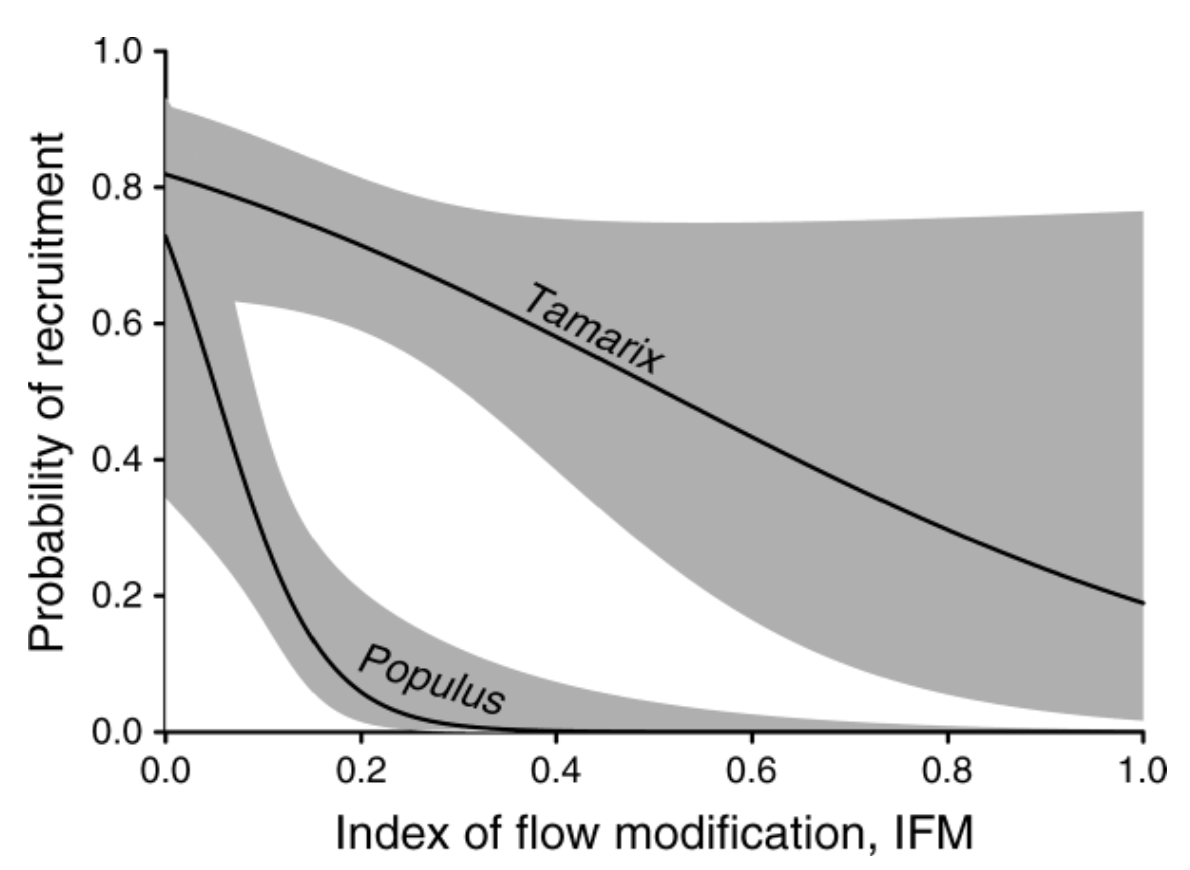
References
Birken, A.S. & Cooper, D.J. (2006) Processes of Tamarix Invasion and Floodplain Development along the Lower Green River, Utah. Ecological Applications, 16, 1103–1120.
Bureau of Reclamation. (2012) Colorado River Basin Water Supply and Demand Study. Online at: http://www.usbr.gov/lc/region/programs/crbstudy.html
Colautti, R.I. & MacIsaac, H.J. (2004) A neutral terminology to define “invasive” species. Diversity and Distributions, 10, 135–141.
Daehler, C.C. & Carino, D.A. (2000) Predicting Invasive Plants: Prospects for a General Screening System Based on Current Regional Models. Biological Invasions, 2, 93–102.
Dewine, J.M. & Cooper, D.J. (2008) Canopy shade and the successional replacement of tamarisk by native box elder. Journal of Applied Ecology, 45, 505–514.
Gaskin, J.F., Birken, A.S. & Cooper, D.J. (2012) Levels of novel hybridization in the saltcedar invasion compared over seven decades. Biological Invasions, 14, 693–699.
Howarth, F.G. (1991) Environmental Impacts of Classical Biological Control. Annual Review of Entomology, 36, 485–509.
Jotter, L. & Clover, E.U. (1944) Floristic studies in the canyon of the Colorado and tributaries. American Midland Naturalist, 32, 591–642.
Keller, R.P., Lodge, D.M. & Finnoff, D.C. (2007) Risk assessment for invasive species produces net bioeconomic benefits. Proceedings of the National Academy of Sciences of the United States of America, 104, 203–7.
Kui, L. & Stella, J.C. (2016) Fluvial sediment burial increases mortality of young riparian trees but induces compensatory growth response in survivors. Forest Ecology and Management, 366, 32–40.
Lesica, P. & DeLuca, T.H. (2004) Is tamarisk allelopathic? Plant and Soil, 267, 357–365. Louda, S.M. (1997) Ecological Effects of an Insect Introduced for the Biological Control of
Weeds. Science, 277, 1088–1090.
McGeoch, M.A., Butchart, S.H.M., Spear, D., Marais, E., Kleynhans, E.J., Symes, A., Chanson, J. & Hoffmann, M. (2010) Global indicators of biological invasion: Species numbers, biodiversity impact and policy responses. Diversity and Distributions, 16, 95–108.
McGuire, R.D. (2015) Transpiration , Growth And Survival Of Native Riparian And Introduced Saltcedar Trees In Mixed Stands On The San Pedro River, U.S.A. University of Arizona. PhD disseration.
Merritt, D.M. & Poff, N.L. (2010) Shifting dominance of riparian Populus and Tamarix along gradients of flow alteration in western North American rivers. Ecological Applications, 20, 135–152.
Perkins, D.W., Scott, M.L. & Naumann, T. (2015) Abundance of Invasive, Non-Native Riparian Herbs in Relation to River Regulation. River research and applications. DOI: 10.1002/rra.2981
Pimentel, D., Zuniga, R. & Morrison, D. (2005) Update on the environmental and economic costs associated with alien-invasive species in the United States. Ecological Economics, 52, 273–288.
Sher, A.A. & Marshall, D.L. (2003) Seedling competition between native Populus deltoides (Salicaceae) and exotic Tamarix ramosissima (Tamaricaceae) across water regimes and substrate types. American Journal of Botany, 90, 413–422.
Sher, A. & Quigley, M.F. (2013) Tamarix: A Case Study of Ecological Change in the American West. Oxford University Press, New York, N.Y.
Stromberg, J.C., Chew, M.K., Nagler, P.L. & Glenn, E.P. (2009) Changing perceptions of change: The role of scientists in Tamarix and river management. Restoration Ecology, 17, 177–186.
Tamarisk Coalition. (2015) Tamarisk Beetle. URL http://www.tamariskcoalition.org/programs/tamarisk-beetle [accessed 2 March 2016]
Tracy, J.L. & Robbins, T.O. (2009) Taxonomic revision and biogeography of the Tamarix-feeding
Diorhabda elongata (Brullé, 1832) species group (Coleoptera: Chrysomelidae: Galerucinae: Galerucini) and analysis of their potential in biological control of Tamarisk. Zootaxa, 2101, 152.
Yin, C.H., Feng, G., Zhang, F., Tian, C.Y. & Tang, C. (2010) Enrichment of soil fertility and salinity by tamarisk in saline soils on the northern edge of the Taklamakan Desert. Agricultural Water Management, 97, 1978–1986.